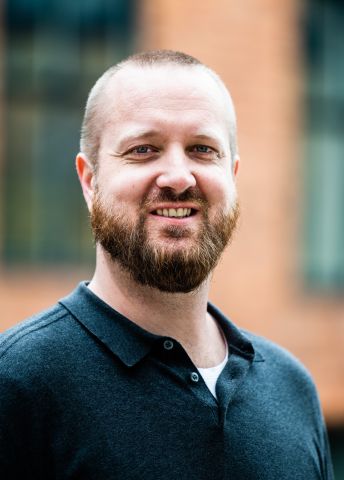
Klas Tybrandt
Associate Professor of Applied Physics
Wallenberg Academy Fellow 2021
Institution:
Linköping University
Research field:
Soft and stretchable bioelectronics
Wallenberg Academy Fellow 2021
Institution:
Linköping University
Research field:
Soft and stretchable bioelectronics
Deep brain stimulation is now an established form of therapy. It involves placing electrodes at selected points in the brain so that short electrical pulses can be used to stimulate the surrounding neurons. Among other things, the method is used to treat patients with Parkinson’s disease, epilepsy, depression and chronic pain.
But the electrodes currently used are hard, which poses a risk of damage to brain tissue, particularly in conjunction with bodily movements. This limits their precision, and if an electrode is displaced, it also loses contact with the right neurons.
Ever since his time as a postdoc, Tybrandt has researched soft and stretchable electronics.
“We’re using methods from materials science to create soft and deformable electrical materials. These can be used in applications that require integration with the body, for examples implants, on-skin patches, and smart clothing,” Tybrandt says.
The aim is to create thin, elastic electrodes that are soft enough to follow the movements of tissues. These soft electrodes will then be able to maintain better contact with the nervous system, which will pave the way for more detailed information transfer.
We’re developing unique soft and elastic microelectrodes for use as implants. We’re very much at the forefront in this field.
As far as deep brain stimulation is concerned, he envisions a new type of electrode consisting of a bundle of ultrathin and stretchable microelectrodes. Once they have been placed in the brain, each microelectrode “uncoils” its arms like an octopus, establishing precise contact with surrounding neurons. Since they are made of a soft and stretchable material, they remain in place despite movements in the tissue.
“Theoretically, this ought to be the ideal solution. We’re using a tiny entry hole and then dispersing the microelectrodes so they have a large contact area and can capture detailed information from adjacent neurons. But to succeed we will have to further develop the electrical materials and find a way to guide the neural probes to the right place.”
Each microelectrode is finer than a human hair. Soft and stretchable, it consists of a network of metal particles that can move in relation to each other.
“Our specialty is developing gold nanowires that are a thousand times finer than human hair. Then we create a network of nanowires encased in silicone rubber. As the silicone deforms, the network does too, and its electrical properties are retained,” Tybrandt explains.
Prototype soft electrodes already exist in the lab. But fabrication of the nanowires and the silicone composite both need to be refined.
Also, Tybrandt’s research team needs to develop a way of guiding the neural probes to the right place in the brain. The solution they favor is to make the tips magnetic. This will enable each probe to be positioned using an external magnetic field.
“But we need a material that can lose its magnetic property over time. It’s not a good idea to have loads of tiny magnetic needles in the brain that might be affected by surrounding magnetic fields. So we’re developing new non-toxic materials whose magnetic properties we can control.”
In order to be absolutely sure that each probe is correctly positioned, it is also necessary to monitor the procedure. Once they are in place the microelectrodes essentially become part of the brain.
“If you insert something that only has a width of 50–100 micrometers in the brain, wounds tend to heal very well. A number of current deep brain stimulation therapies also involve lifelong treatment, so our electrodes need to have a lifespan that is longer than that of the patients,” Tybrandt says.
The concept itself will first be tested in hydrogels, and then in neural tissues in the laboratory. The final stage is to develop the finished electrode for use in living tissue. Ultimate success requires close collaboration with researchers in a number of other fields – medical fields in particular.
“Our primary approach is to develop applications for treating neural damage caused by accidents or disease. We also want to improve existing therapies involving deep brain stimulation.”
Over the longer term there is the prospect of joining up the body’s nervous system with prostheses of various kinds that can then be controlled by the body’s own neural signals. There is also the possibility of connecting the human brain to digital networks or future technologies. Although it is still a long way away, this futuristic idea has enormous potential. Tybrandt elaborates:
“To succeed we have to traverse the full spectrum – from theory and simulation via materials synthesis to development of new applications. It’s extremely broad, but that’s also what makes it so exciting. And many of the major strides currently being made in research are taking place at the interface between different fields.”
Text Magnus Trogen Pahlén
Translation Maxwell Arding
Photo Thor Balkhed
“