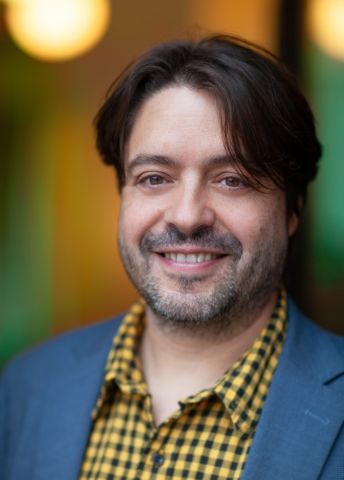
Philipp Schlatter
Professor of Fluid Mechanics
Wallenberg Academy Fellow, prolongation grant 2018
Institution:
KTH Royal Institute of Technology
Research field:
Turbulence
Wallenberg Academy Fellow, prolongation grant 2018
Institution:
KTH Royal Institute of Technology
Research field:
Turbulence
Wind tunnels play a central part in the development of aircraft and other vehicles. Large fans blow air over vehicles covered with sensors, the aim being to find the best and most fuel-efficient design.
But the tunnels are costly to build and use, and the sensors are sensitive. Simulating tests on a computer is a more cost-effective approach.
“When we simulate the trials virtually, we have more control, and it is simpler to adjust the many variables – everything from velocities to temperatures,” Schlatter says.
But virtual simulations pose numerous challenges, the main one being the turbulence itself. Schlatter explains:
“These days turbulence is one of the trickiest areas of classical physics.”
Turbulence is defined as irregular fluctuations occurring at random when a gas or a liquid reaches high speed. Hundreds of thousands of interacting eddies form in the chaotic flow.
In a simulation, each eddy is computed mathematically. The computations are based on a grid of points describing the surface of an aircraft wing, for instance. The behavior at each point, or eddy, is described with the help of equations that result in more or less exact mean values. A huge number of computations are required, making great demands of methods and computers alike.
Schlatter’s goal is to simulate an aircraft wing about two meters long, traveling about two hundred kilometers an hour. A similar simulation of a whole aircraft wing moving at that speed for one second would require all computing power available on Earth. But he does not think that is ever going to happen.
“It’s more important to develop current methods so we can learn more about how the eddies interact. This will pave the way for new turbulence models that yield the same information without simulating a whole aircraft.”
Schlatter’s research team has already succeeded in simulating a part of an aircraft wing that moves at approximately the same speed as a glider. The simulation has been validated experimentally in the wind tunnel at KTH Royal Institute of Technology.
“We showed that we could do the same thing virtually as in a ten-meter long and ten-meter-high wind tunnel. We’re moving on to simulate the very largest wind tunnels in the world.”
Here, classical calculation methods are key, even though developments in AI and machine learning have also been helpful.
“We can use machine learning, for instance, to collate data from hundreds of thousands of eddies, so we can see the importance of each eddy.”
Machine learning can also help increase the accuracy of the simulation by predicting the points at which the computations need to be more detailed.
The research is also benefiting from the new supercomputer initiatives under way in Europe. These include Linköping University’s new supercomputer Berzelius, which has come about thanks to funding from Knut and Alice Wallenberg Foundation. This will be joined by the new Dardel supercomputer, which will be ready for use at KTH Royal Institute of Technology in autumn 2021.
But the real flagship computer is Finnish LUMI, which will be one of the world’s most powerful supercomputers. It is part of the EU EuroHPC project, which is building new supercomputers around Europe.
“LUMI will give our research a real boost. But we will have to adapt our code to the processors that LUMI uses,” Schlatter explains.
LUMI will be part of the supercomputer center in Kajaani, where methods and codes developed within the scope of Schlatter’s research are already in use.
The methods used to simulate turbulence will have a broad spectrum of applications, since turbulence occurs everywhere in nature – in the kitchen when we cook, and in district heating pipes, water and sewage systems.
“This is what makes fluid mechanics so exciting. Findings can be applied in virtually all fields, including medicine, where we can simulate the spread of an airborne disease such as Covid-19.”
“This grant is important because it enables me to develop fundamental methods – a process for which it’s difficult to obtain funding.”
KTH has long been pre-eminent in fluid mechanics, which was why Schlatter want to work there.
“In my family there was never any doubt that I would study for a PhD – the question was in what subject. It was a privileged position, but also very much performance-oriented.”
When he began his studies he chose mathematics, but lost interest because it was mostly about proving solutions without calculating the results.
“It’s important for me to work towards a goal, like simulating a given wing profile. But it doesn’t have to result in an industrial application. It’s more important that the knowledge and methods are disseminated and used.”
At present he is halfway to his goal of simulating a whole wing at cruising pace. But the engineer in him doesn’t find it hard to set new goals in his day-to-day life – not even when he is building Duplo with his daughter.
“Duplo can be quite interesting from a structural viewpoint: how to build something stable. I’ve been struck by the idea of programming a method to simulate different optimal ways of building.”
Text Magnus Trogen Pahlén
Translation Maxwell Arding
Photo Magnus Bergström