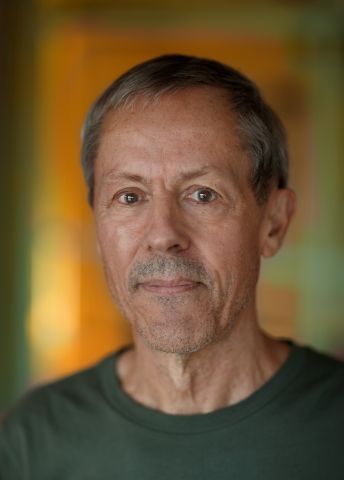
Gunnar von Heijne
Wallenberg Scholar
Institution:
Stockholm University
Research field:
Structure and folding of membrane-bound proteins
Wallenberg Scholar
Institution:
Stockholm University
Research field:
Structure and folding of membrane-bound proteins
Every function in our bodies, from breathing to thinking, is controlled by proteins. These molecules are long chains of amino acids that are strung together in the cell’s protein factories – the ribosomes – based on blueprints from our DNA.
But the chain must be correctly folded if the protein is to work properly. If misfolding occurs, sticky clumps may form. These are associated with diseases such as Alzheimer’s, Parkinson’s and certain cancers.
Researchers have long studied protein folding outside the cell, in test tubes, by first unfolding complete proteins and then allowing them to regain their structure. But this method differs from the way the cell works in real life. Proteins often fold as they emerge, amino acid by amino acid, from ribosomes.
von Heijne and his team have developed their own method, called “Force Profile Analysis” (FPA), which provides radical new insights into the protein folding process.
“FPA enables us to follow the process step by step, and obtain a highly detailed picture of how proteins are built and attain their final form.”
One advantage is that FPA works for two distinct protein worlds – membrane proteins and water-soluble proteins.
“Completely different techniques are often used, but we now have a tool that is equally effective when used on both types,” explains von Heijne.
The method involves use of special amino acid sequences called “arrest peptides,” which can temporarily stop protein fabrication in the ribosome. The researchers place these arrest sequences at strategic points in the protein chain, enabling them to measure the pulling force exerted when the protein begins to fold in the ribosome’s exit tunnel.
The readings obtained enable the researchers to analyze and understand when and how proteins assume their functional form. Among other things, the research shows that the size of the protein impacts the folding process.
“But there are also challenges in interpreting data and understanding exactly what each measurable force signal means at the molecular level.”
The researchers are also using other techniques such as cryoelectron microscopy and molecular dynamics, a computer simulation method, to piece together a complete “film” of how the proteins gradually take shape.
von Heijne has devoted much of his long research career to the study of membrane proteins. They ensure the cell receives the substances and signals it needs and that it can communicate with its surroundings. Some membrane proteins act as the cell’s “gatekeepers.”
As their name suggests, membrane proteins are embedded in the cell membrane – the cells’ protective layer. Part of the research aims to gain an understanding of the processes involved when membrane proteins are drawn into the cell membrane, and how they are constructed to operate in the fat-rich environment.
Some amino acids in the protein thrive in a fatty environment rather than in water, and are thereby drawn into the membrane. But if there are charged hydrophilic amino acids in the wrong place, the process may be impaired.
New discoveries have shown that individual amino acids, particularly those that are electrically charged, play a key role and impact the way a membrane protein is pulled into the cell membrane, and how the protein then functions.
It would be extremely interesting to take a closer look at medical angles, such as protein misfolding, in human diseases, or proteins that do not fold at all, but may nevertheless have a function even though they don’t have a folded structure.
“This is particularly important for charge-sensitive ion channels in neurons. Charged amino acids in those channels enable proteins to detect changes in the voltage over the membrane. This in turn enables the protein to open and close a channel for ions at exactly the right time,” explains von Heijne.
Work to date has very much focused on the E. coli bacterium. von Heijne now wants to transpose the method to yeast cells. Yeast is a model system that resembles human cells, and may enable the research to make major strides.
The idea is to study more advanced, cell biological processes. One such process is the way proteins are pulled into organelles – the cell’s counterpart to the organs in our body.
“Yeast offers a huge genetic toolbox – it’s possible to examine how mutations in different transport machineries impact the protein capture in organelles,” says von Heijne.
This new knowledge may ultimately play a part in applications, for example drugs that can impact protein function, or tailored proteins in the biotech field.
This specialty, which scientists call “cotranslational folding,” is young and growing, as von Heijne explains:
“It’s a relatively narrow field, but it’s growing rapidly. Several labs are developing their own methods. We’re glad our technique is fairly simple and is already being used by other research teams.”
Text Nils Johan Tjärnlund
Translation Maxwell Arding
Photo Magnus Bergström