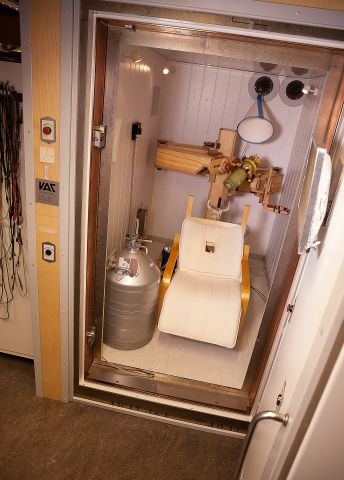
Project Grant 2014
Nanoscale superconducting devices for a closer look at brain activity
Principal investigator:
Dag Winkler, Professor of Physics
Co-investigators:
Chalmers University of Technology
Thilo Bauch
University of Gothenburg
Mikael Elam
Johan Wessberg
Karolinska Institutet
Martin Ingvar
Institution:
Chalmers University of Technology
Grant in SEK:
SEK 34.4 million over five years
“This project is one of the most exciting things I have done in my research career,” says Dag Winkler, Professor of Physics at Chalmers University of Technology.
He and Justin Schneiderman, Associate Professor of Medical Technology at the University of Gothenburg and MedTech West, enthusiastically describe the interdisciplinary research project they are jointly coordinating. The aim is to develop a more sensitive sensor system for magnetoencephalography (MEG), an advanced instrument that measures brain activity.
The project brings researchers in physics that are developing superconducting nanoscale components together with brain researchers in neuroscience and physiology. The research is a continuation of earlier progress in these fields. Thanks to funding from the Knut and Alice Wallenberg Foundation, the process of combining the research teams has gained new momentum.
“The grant enables us to link up the research here at Chalmers and Karolinska Institutet with that being conducted at the University of Gothenburg and the Sahlgrenska Academy. This means that we can create a strong Swedish and Nordic hub for research into sensor technology and brain imaging,” Dag says.
The SQUID senses magnetic fields
MEG uses a very expensive instrument that can record the magnetic fields produced by electric currents generated by neurons in the brain. Such systems are found at some specialist hospitals and are used both for research and clinical examinations, e.g., when preparing for surgical brain interventions, and in diagnosing epilepsy and dementia.
There is only one full MEG system in Sweden today. It was bought by Karolinska Institutet with funding from the Knut and Alice Wallenberg Foundation, and is operated by NatMEG – the Swedish National Facility for Magnetoencephalography– with which the project is collaborating.
“By comparing the new sensor technology with this state-of-the-art MEG system while learning more about the clinical and research needs, we will be able to further refine our components,” explains Justin Schneiderman.
Dag explains that the sensors that record the brain’s electrical activity inside the MEG use a highly sensitive detector called a SQUID – Superconducting Quantum Interference Device. Chalmers has a long tradition in the field of superconducting circuits and applications of superconductivity.
“We have now developed a highly sensitive SQUID that will make MEG both less expensive and simpler to use. We hope that we will be able to record brain activity with a higher resolution, like a camera with more pixels.”
Closer to the skull
Superconductivity means that current can pass without resistance through certain materials. The phenomenon occurs below a certain critical temperature. Current MEG technology uses low-temperature SQUIDs, which must be cooled down to -269°C.
“We are the first research team in the world to show that high-temperature SQUIDs, with a signal-to-noise ratio similar to standard MEG systems, can be used to record spontaneous brain activity. We have also registered signals from the brain that could not be seen before using other technology,” Dag says.
“These high-temperature SQUIDs that may be based on nanothreads only need cooling down to -196°C. This yields a number of advantages,” Justin explains while he uses Dag to show how brain activity is recorded in the lab. A magnetically screened room has been installed so that the experiments will not suffer interference from environmental magnetic fields.
“Our SQUIDs require less thermal insulation, which means we can get closer to the head. This is important, since magnetic fields fade away quite quickly with distance. In fact, we can clearly see that the signals are even stronger than we had thought,” Justin adds.
It is a tremendous advantage to be able to cool the new SQUIDs down using liquid nitrogen. It is less complicated and entails substantially lower costs than the helium that state-of-the-art MEG systems require.
Dust-free manufacture
A number of floors down from the measurement lab lies Chalmers’ advanced clean room, largely funded by the Foundation. SQUIDs and other nanoscale electronic components are made here under completely dust-free conditions. The researchers will be endeavoring to learn more about quantum phenomena in the high-temperature superconducting nanomaterials, and will also be developing new nano-SQUIDs.
“One approach is to have several of these SQUIDs in a kind of cluster to make it easier to connect the magnetic field to them,” Dag explains.
One major challenge in the project is to stitch together the technical research with its applications in the medical field. For instance, the technique will be used at the University of Gothenburg in studies on how the body is affected by stress. A further application will be to interpret the signals generated when we touch something – how the brain understands the difference between rough and smooth, for example.
“It feels as though we are well on the way to piecing together the work being done by the different research teams; there is a lot of positive energy in the project,” Dag concludes.
Text Susanne Rosén
Translation Maxwell Arding
Photo Magnus Bergströ