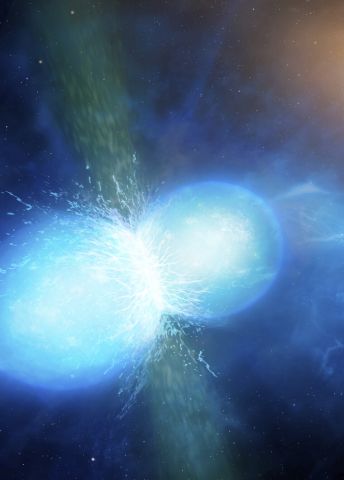
Project Grant 2020
Creation of heavy elements in neutron-star mergers
Principal investigator:
Associate Professor Andreas Heinz
Co-investigators:
Chalmers University of Technology
Håkan Johansson
Thomas Nilsson
Institution:
Chalmers University of Technology
Grant in SEK:
SEK 29,600,000 over five years
Big Bang – the birth of the universe – created the very lightest elements – hydrogen in particular. All heavier elements in our bodies and around us were later formed by processes in the cosmos. The most common one is the energy process that causes our sun and other stars to shine: in the core of stars small, light atomic nuclei fuse together to form heavier atomic nuclei, releasing huge amounts of energy in the process.
Elements up to nickel and iron can be formed in this way. But heavier ones will only form if atomic nuclei capture free neutrons – particles that are normally found in atomic nuclei themselves (see fact box below).
“Free neutrons are unusual because they are so short-lived. But large numbers of neutrons are liberated at some events in the universe. One example is when a massive star dies, exploding into a supernova,” explains Andreas Heinz, who researches in subatomic physics at Chalmers.
Supernovas not enough
Observations and calculations have indeed shown that flows of neutrons in supernovae give rise to many heavier elements. But as long ago as the 1950s scientists realized that supernovae alone hardly suffice to explain the existence of some heavy elements – among others gold and platinum.
Theoretical physicists therefore postulated a further process for the formation of heavy elements – a rapid neutron capture process known as the r-process (r for rapid). It occurs when atomic nuclei absorb multiple neutrons in rapid succession, and requires an extremely high density of free neutrons, much higher than what occurs in supernova explosions.
“It was suggested that such high neutron flows might be created when two neutron stars collide. Neutron stars are extremely dense – heavier than our sun but only about 25 kilometers in diameter.”
Collision revealed by ripples in space-time
Collisions between neutron stars are unfortunately rare and difficult to detect – they produce very little in the way of characteristic light or radio waves. It was not until 2017 – seven decades after the r-process theory was postulated – that scientists managed to definitely identify two colliding neutron stars. They did so by detecting gravitational waves caused by the collision. Numerous telescopes were then aimed at the collision, and researchers were able to infer a huge flow of neutrons.
“The observations gave strong indications that heavy elements are formed in neutron star collisions. Now it’s time to establish how that happens. The r-process entails thousands of steps via numerous isotopes that no human has ever seen and remains to a large extent unknown,” Heinz says.
He is now leading a research project, funded by Knut and Alice Wallenberg Foundation, with the aim of exploring the role of nuclear fission in the r-process.
In the r-process atomic nuclei capture neutron after neutron at a rapid pace. After a while, the atomic nucleus becomes so unstable that a form of radioactive decay known as “beta decay” occurs, transforming one of the neutrons in the nucleus into a proton. This transforms the atomic nucleus into a slightly heavier element.
Nuclear fission sets an upper limit
After many cycles involving neutron capture and beta decay the atomic nucleus is so big and heavy that it can undergo nuclear fission, that is, split into two lighter elements.
“Fission prevents heavier elements from forming. But no one knows at what point in the r-process or how many times fission enters the picture. Perhaps the atomic nuclei undergo many cycles in which they are first built up and then split, or maybe it only happens once,” Heinz reflects.
A common feature of the atomic nuclei formed in the r-process is that they contain an unusually high number of neutrons. As yet we know very little about how neutron-rich nuclei split. Heinz and his two fellow researchers want to change this by means of innovative experiments at CERN – the European Organization for Nuclear Research. They can do so because the ISOLDE facility for radioactive atomic nuclei at CERN has just been fitted with a completely new type of instrument that enables researchers to measure the conditions under which highly unstable atomic nuclei split.
As part of the project, the researchers will be carefully measuring the energy required to split different heavy atomic nuclei such as francium, radon and bismuth, along with the probability that fission will occur. This information can then be used to develop models that help the research community to study and understand the role played by nuclear fission in the r-process.
“The goal of this research is essentially to answer a fundamental question about our existence: where do we and the world around us come from?”
Text Ingela Roos
Translation Maxwell Arding
Photo Björn Jonson, Andreas Heinz, University of Warwick/Mark Garlick, ESO/L. Calçada/M. Kornmesser, Anna-Lena Lundqvist
Facts about atomic nuclei
Atomic nuclei consist of two types of particles: positively charged protons and electrically neutral neutrons.
The number of protons in the atomic nucleus determines the chemical element to which it belongs.
Isotopes are atoms of the same chemical element, but with different numbers of neutrons in the atomic nucleus.
In beta decay of neutron-rich nuclei, a neutron is transformed into a proton, and an electron and an “anti-electron neutrino” are emitted.
In nuclear fission, a nucleus splits into two lighter nuclei – either spontaneously or after absorbing a neutron.