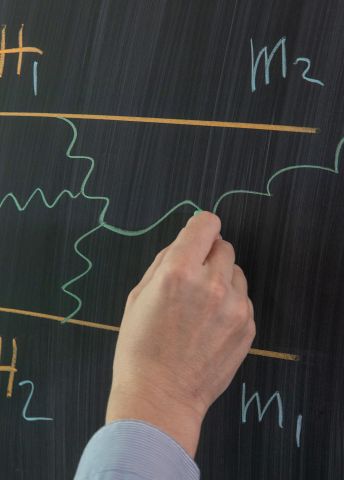
Project Grant 2018
From Scattering Amplitudes to Gravitational Waves
Principal investigator:
Henrik Johansson
Co-investigator:
Nordita – Nordic Institute for Theoretical Physics
Paolo Di Vecchia
Institution:
Uppsala University
Grant in SEK:
SEK 19,100,000 over five years
It caused quite a stir among the scientific community when the news was announced with great fanfare in February 2016. Using an upgraded LIGO detector, researchers had discovered gravitational waves as traces left behind after the collision of two black holes. The news broke almost exactly one hundred years after Einstein’s prediction, and provided an insight into processes taking place billions of light years away.
Henrik Johansson is a researcher in particle physics at Uppsala University.
“It was a fantastic discovery. Those familiar with all the details of the experiment realized that something special was about to happen, but it came as a surprise to the rest of the research community.”
Black holes yield surprises
The breakthrough came after decades of attempts to confirm Einstein’s theories on gravitational waves. Increasingly advanced detectors and mirrors have been built over the years. Equipment now exists that is capable of measuring with exceptional accuracy how the trajectory of a laser beam shrinks and is stretched once again as a gravitational wave passes by.
“Many people were surprised that the black holes were much larger than expected. It had been thought that they should be a few times heavier than the sun, but their mass was 50 to 60 times greater than that of the sun.”
The scientists who discovered gravitational waves were awarded the Nobel Prize in 2017. Their discovery has opened a new window to the universe. Further revelations are expected over the next few years.
“We’ll be able to learn more about black holes and gain a better understanding of neutron stars by learning more about the matter inside them. We might also be able to see the gravitational waves from the very origins of the universe, right after Big Bang.”
More efficient methods
But if we are to benefit from the new experimental opportunities, the theoretical physics will also have to evolve. Funded by Knut and Alice Wallenberg Foundation, Johansson is leading a project to develop new, efficient analytical methods for theoretical calculations in fundamental physics. Over the past few years he has established one of the world’s leading research environments in this field.
“The project is not just about gravity; it also involves particle physics and string theory. There is arguably quite a difference between calculating collisions of elementary particles – which are described by quantum mechanical scattering amplitudes – and colliding black holes, which are described by the theory of general relativity. But the underlying mathematical description is almost identical,” Johansson says.
He made a breakthrough in the field about a decade ago, when he and two colleagues showed that it is possible to study gravitational physics using the same mathematical details that is used to describe microscopic processes in particle physics, through what is known as Feynman diagrams. The aim is now to simplify the calculational methods that directly or indirectly make use of these diagrams.
“The calculations that used to keep a PhD student busy for several years can now be done in less than a minute on the blackboard.”
Dramatic simplification
A benchmark study for the newly developed methods was given by a calculation of a gravitational amplitude derived from a supergravity theory. Here, the researchers base their approach on Einstein’s theory of general relativity, but add extra particles to achieve something known as supersymmetry.
“Even though we have more particles than in Einstein’s theory, those extra particles simplify the calculations because they eliminate certain terms. This enables us to test-run complicated calculations before we look at Einstein’s theory.”
The researchers succeeded in expressing the amplitude using 750 diagrams, each having fewer than 10,000 terms. It may sound a lot, but not compared with standard methods. Johansson elaborates:
“If I had used the Feynman diagrams that directly come from the theory of general relativity, as done in standard approaches, I would have needed to use more than 10,000 diagrams, each one having more than a million terms – a truly impossible calculation! So we managed to do the impossible…”
A new level of theoretical physics
But further development of more efficient calculation methods is needed. The new experimental gravitational wave detectors have increasingly high resolution and are more accurate. This means that the current theoretical level is insufficient.
“We have to reach the sixth order of what we call ‘perturbation theory’. And right now we haven’t quite reached half way there. And the higher the order, the harder it gets. So researchers, postdocs and PhD students will have to buckle down and try to improve these calculations.”
The theoretical predictions will have to be improved if there is to be any point in improving the design of the experiments.
“Unless theoreticians do their job and perform the calculations, there’s no point in improving the experiments,” Johansson comments.
Text Nils Johan Tjärnlund
Translation Maxwell Arding
Photo Magnus Bergström